1. What is Radioactivity?
We can define radioactivity as the spontaneous disintegration of unstable atomic nuclei with the emission of alpha (α), beta (β), or gamma (γ) radiations and the release of energy. Additionally, we can say that radioactivity is a natural process that occurs in certain elements, such as uranium and thorium, and their unstable nuclei.
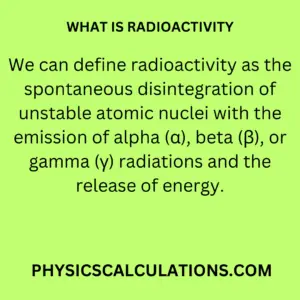
These radioactive elements emit radiation in the form of alpha, beta, and gamma radiations, leading to a transformation into stable nuclides through nuclear decay.
Understanding Radioactivity
At its essence, radioactivity can be defined as the innate propensity of atomic nuclei to undergo spontaneous disintegration, transmuting unstable nuclei into more stable configurations. This remarkable natural phenomenon is governed by the strong and weak nuclear forces, which dictate the behaviour of the fundamental building blocks of matter, the protons, and neutrons within atomic nuclei.
Unstable atomic nuclei, those teetering on the edge of stability due to an imbalance between protons and neutrons, yearn to achieve equilibrium. Their means of achieving this equilibrium involves a first-order process known as radioactive decay. In this process, these unstable nuclei shed excess energy and particles to transition to more stable configurations.
A very important concept in radioactivity is half-life. This measure signifies the time it takes for half of a given quantity of radioactive material to decay. Half-life varies widely among different isotopes, and its determination is central to various applications in nuclear physics, chemistry, and even archaeology.
a. Atomic Nuclei
At the heart of the atom lies the nucleus, a minuscule yet immensely significant region where protons and neutrons cluster, held together by the formidable grip of nuclear forces. The nucleus defines the identity of an element, dictating its atomic number and mass number. Yet, not all atomic nuclei are created equal. Some are inherently unstable, and it is within these fragile entities that the magic of radioactivity unfolds.
b. Unstable Nuclei
The crux of radioactivity lies in the realm of unstable atomic nuclei. These nuclei are inherently predisposed to change, to evolve into more stable configurations. This inherent instability is what sets the stage for the wondrous processes of radioactive decay.
c. First-Order Process
Radioactive decay is inherently a first-order process, characterized by its spontaneity. It is a phenomenon that defies predictability; the exact moment when a radioactive nucleus will decay remains uncertain. This unpredictability is the essence of radioactivity and makes it all the more intriguing.
d. Half-life
To fully understand the secrets of radioactivity, one must comprehend the notion of half-life. It is the time it takes for half of a collection of radioactive nuclei to decay. The concept of half-life is indispensable in understanding the kinetics of radioactive decay.
e. The Energetics and Kinetics of Radioactivity
One cannot delve into the universe of radioactivity without understanding the energy dynamics at play. Every decay process leads to the release of energy, manifesting as kinetic energy of the emitted particles and photons. This energy release is intrinsic to the workings of nuclear power and radiometric dating techniques.
Intriguingly, the decay rate of radioactive materials follows an exponential decay pattern, an aspect central to the concept of half-life. The precise determination of half-life not only reveals the stability of a given isotope but is invaluable in radiometric dating methods, illuminating the chronology of geological and archeological artifacts.
f. Variability in Decay: Temperature and Pressure
While radioactive decay is generally predictable on a statistical level, it’s not entirely immune to external influences. Temperature and pressure can influence the decay rate. This intriguing feature adds a layer of complexity to the study of radioactivity.
g. Representation of Radioactive Species
Understanding and representing radioactive species involves a specific notation, often highlighting the element’s atomic number and mass number. This notation captures the essence of radioactivity and plays a fundamental role in the nuclear sciences.
2. Types of Radioactive Particles
There are three types of radioactive particles: alpha, beta, and gamma particles. Alpha particles consist of two protons and two neutrons, making them the heaviest of the three particles. Beta particles are electrons that are emitted from the nucleus. Gamma particles are high-energy photons that are emitted from the nucleus. These particles have different properties that make them unique.
a. Alpha Decay
In this phenomenon, alpha decay entails the ejection of alpha particles from the nucleus. Alpha particles consist of two protons and two neutrons, essentially forming helium nuclei. The contributions of the esteemed physicist Ernest Rutherford significantly illuminate this type of decay.
b. Beta Decay
The second of our trio, beta Decay, involves the emission of beta particles, which are essentially electrons (in the case of beta-minus decay) or positrons (in the case of beta-plus decay). The conversion of a neutron into a proton or vice versa is the essence of beta decay. The process unveils the intricate balance of nuclear forces.
c. Gamma Decay
To complete our trio, we now look at Gamma Decay, which involves the nucleus releasing high-energy photons known as gamma rays. These emissions signify a transition within the nucleus to a lower energy state. Gamma rays are a form of ionizing radiation, capable of penetrating matter and vital in various scientific and medical applications.
3. Properties of Alpha, Beta, and Gamma Particles
Alpha particles are the heaviest of the three particles and have a positive charge. Therefore, we can stop them with a piece of paper or the skin, making them relatively safe to handle. Also, ingesting or inhaling alpha particles can cause damage to living tissues. Beta particles are lighter and have a negative charge. They can penetrate through materials like aluminum foil and can cause harm to living organisms if they enter the body through inhalation, ingestion, or absorption through the skin. Gamma particles are the most penetrating and have no charge. They require a thick layer of lead or concrete to stop them. Thus, they can cause damage to cells and tissues when they pass through the body.
4. Units of Radioactivity
Introduction to Units of Radioactivity – The world of nuclear physics requires precise measurements, and understanding the units of radioactivity is paramount. Two primary units, the Curie (Ci) and the Becquerel (Bq), are central to quantifying radioactivity.
Subunits of Radioactivity – In the realm of radioactivity, precision is of the essence. Smaller units, such as the picocurie (pCi), nanocurie (nCi), microcurie (µCi), and millicurie (mCi), provide a more detailed scale for measurement. Each unit offers unique insights into the intensity of radioactivity.
Historical Perspective: The Curie – The Curie, named in honour of Marie Curie, a pioneering physicist, and chemist, was an early unit used to measure radioactivity. Its historical significance is a testament to the groundbreaking work in the field.
The International Standard: Becquerel (Bq) – In the modern world of nuclear physics, the Becquerel (Bq) serves as the international standard for measuring radioactivity. Named after Henri Becquerel, it quantifies the number of disintegrations per second.
Radioactivity is not confined to esoteric scientific laboratories; it permeates the world around us. Our environment contains both naturally occurring radioactive elements and isotopes, known as primordial sources, such as uranium and thorium. These elements contribute to background radiation and geological processes, playing an instrumental role in the evolution of life on Earth.
Notably, the quest for knowledge has led to the creation of artificial isotopes through nuclear reactions. The world of nuclear physics has ventured into the realm of induced radioactivity, manipulating the atomic nucleus to serve scientific, medical, and industrial purposes.
a. Natural Radioactivity
Natural radioactivity refers to the radioactivity that occurs naturally in the environment, including the radioactive decay of elements like uranium and thorium. The decay of uranium produces radon gas. This is a naturally occurring radioactive gas that can accumulate in homes and other buildings, leading to increased exposure to ionizing radiation.
b. Artificial Radioactivity
Artificial radioactivity refers to the induced or man-made creation of radioactive isotopes through nuclear reactions. Unlike naturally occurring radioisotopes, which exist in the environment, artificial radioisotopes are produced intentionally in laboratories or nuclear facilities. This process involves bombarding stable isotopes with particles, such as protons or neutrons, to alter their nuclear composition. The resulting radioactive isotopes find applications in various fields, including medicine, industry, and research. Artificial radioactivity allows scientists to tailor isotopes with specific properties for use in diagnostic imaging, cancer treatment, sterilization processes, and scientific investigations. While offering valuable tools, the careful management and disposal of artificially produced radioactive materials are essential to mitigate potential environmental and health risks.
5. Radioactive Elements
Radioactive elements are those that have an unstable nucleus and undergo radioactive decay. Additionally, Uranium and thorium are two naturally occurring radioactive elements that are commonly found in the Earth’s crust. Uranium-238 is the most abundant naturally occurring isotope of uranium, while thorium-232 is the most common isotope of thorium. We can use both elements in nuclear power generation and other applications.
6. Nuclear Decay
Radioactive decay is the process by which unstable atomic nuclei emit particles and energy in order to achieve a more stable configuration. There are several types of radioactive decay, including alpha decay, beta decay, and gamma decay. During alpha decay, the nucleus emits an alpha particle, reducing the atomic number by two and the atomic mass by four. Beta decay involves the emission of a beta particle, which can be either an electron or a positron, from the nucleus. Gamma decay is the emission of gamma rays from the nucleus.
a. Radii and Cross-Sectional Areas of Nuclei: Peering Inside the Nucleus
Atomic Nuclei: The Subatomic Strongholds – Atomic nuclei, as the densest part of the atom, are filled with protons and neutrons bound by nuclear forces. To gain insight into the fascinating world of nuclear physics, one must understand the composition and structure of atomic nuclei.
Size and Radii of Atomic Nuclei – The size of atomic nuclei is minuscule compared to the atom as a whole, yet it significantly impacts the behaviour of atoms. Understanding the size and radii of atomic nuclei provides a key to unlocking their properties.
Significance of Nuclear Cross-Sectional Areas – Nuclear cross-sectional areas play a vital role in understanding the probability of interactions between atomic nuclei and subatomic particles. These areas are central to nuclear reactions and are essential for nuclear physicists.
Comparison with the Size of Atoms – When delving into nuclear physics, it becomes evident that atomic nuclei are incredibly small compared to the overall size of an atom. This disparity reveals the true nature of matter, where most of the mass is concentrated in the nucleus.
b. Mass Concentration in Nuclei
Mass Concentration within Nuclei – The concentration of mass within atomic nuclei is astonishing. Protons and neutrons, which constitute atomic nuclei, are nearly two thousand times heavier than electrons that orbit the nucleus. The nucleus holds the key to understanding mass-energy equivalence.
Comparing Masses: Nucleons vs. Electrons – The comparison between the masses of nucleons (protons and neutrons) and electrons is staggering. Nucleons are considerably more massive than electrons, a fact that highlights the significance of nuclear physics in the broader realm of particle physics.
Mass-Energy Equivalence: E=mc² – Albert Einstein’s revolutionary equation, E=mc², serves as a cornerstone in nuclear physics. In the context of nuclear reactions, even a small change in mass results in a substantial release of energy, fundamentally altering the nuclear landscape.
c. Branching Decay Processes: The Complexity of Transformations
Explanation of Branching Decay Processes – The world of radioactivity often involves complexity beyond a simple, single-step decay. Branching decay processes occur when a parent radionuclide has the capability to undergo multiple transformation pathways.
Total Transformation Constants – Calculating the total transformation constants for branching decay processes is an intricate task. Yet, these constants are fundamental to understanding the fate of radioactive species and their transformations.
Example of Branched Decay in 40K – Potassium-40 (40K), a naturally occurring radioactive isotope, offers a compelling example of branching decay. The isotope’s journey through various transformation pathways serves as an illuminating case study.
7. Ionizing Radiation
Ionizing radiation is a type of radiation that has enough energy to ionize atoms or molecules, leading to the formation of ions. This can cause damage to living tissues, leading to health problems like cancer and genetic mutations. Examples of ionizing radiation
Gamma radiation differs from the other two types of radiation because it doesn’t consist of particles. Instead, it’s a form of energy that’s released by the unstable nucleus in the form of electromagnetic waves. Gamma radiation is very powerful and has a lot of energy, making it the most dangerous of the three types of radiation. Unlike alpha and beta radiation, gamma radiation can penetrate most materials, including concrete and lead.
Now that you know about the different types of radiation, let’s take a closer look at what radioactivity is. Radioactivity is the process by which an unstable nucleus emits radiation in order to become more stable. Radioactivity can occur naturally, as with radioactive elements such as uranium and thorium, or it can be induced artificially, as with nuclear reactors and nuclear bombs.
Heavy Ion Radioactivity: Unveiling the Extraordinary
a. Overview of Heavy Ion Radioactivity – While alpha decay may be a familiar concept, the domain of heavy ion radioactivity reveals a fascinating layer of nuclear instability. These enigmatic emissions provide profound insights into the behavior of atomic nuclei.
b. Prediction and Experimental Evidence – The existence of heavy ion radioactivity was initially a matter of theoretical prediction. However, through meticulous experimentation, the evidence for heavy ion radioactivity became compelling.
c. Cluster Emitters and Nuclear Stability – Heavy ion radioactivity often involves the emission of clusters of nucleons. This unique phenomenon sheds light on the precarious equilibrium of atomic nuclei and challenges the conventional understanding of nuclear stability.
d. Comparison with Alpha Decay – While both heavy ion radioactivity and alpha decay involve the emission of charged particles, they exhibit crucial differences. Understanding these disparities is central to the study of nuclear phenomena.
e. Notable Examples of Heavy Ion Radioactivity – The realm of heavy ion radioactivity introduces us to a variety of fascinating examples, including the decay of carbon-14 and other isotopes.
These examples serve as windows into the intricate dance of nuclear decay.
8. Charge, Mass, and Penetration Power of the Elements
In order to better understand the properties of the different types of radiation, let’s take a look at the following table:
Particle | Charge | Mass | Penetration Power |
Alpha | +2 | High | Low |
Beta | -1 | Low | Medium |
Gamma | 0 | None | High |
As you can see, alpha particles are the heaviest of the three types of radiation and have the lowest penetration power, meaning they can be stopped by a piece of paper or even the outer layers of human skin. Beta particles are lighter and have medium penetration power, meaning they can pass through materials such as wood and plastic, but are stopped by materials such as metal. Gamma radiation, on the other hand, has no mass and the highest penetration power, meaning it can penetrate most materials.
9. Effects of Radioactive Particles
Radioactive particles can have harmful effects on living organisms, including humans. When alpha and beta particles enter the body, they can cause damage to cells and tissues, leading to cancer or other health problems. Gamma rays can also cause damage to cells and tissues but can pass through the body, affecting internal organs. Exposure to ionizing radiation can also cause genetic mutations and birth defects.
10. Protecting Yourself from Radioactivity
To protect yourself from the harmful effects of radioactivity, it is important to take appropriate safety measures. This includes wearing protective clothing, using shielding materials like lead, and avoiding exposure to radioactive sources. Following safety guidelines and regulations when handling radioactive materials is also important.
11. Practical Applications of Radioactivity
The essence of radioactivity extends far beyond the realm of scientific curiosity. Its practical applications find resonance in diverse fields, offering a spectrum of advantages. In medicine, radioisotopes are harnessed for diagnostic imaging and cancer treatments. They act as radioactive probes, enabling the investigation of cellular processes and aiding in the identification of specific tissues.
Furthermore, radioactivity plays a pivotal role in sterilization processes, a key component of medical device production and food preservation. It is indispensable in the oil and gas industry for tracing fluid flow in reservoirs. In the realms of archeology and geology, radiocarbon dating enables the determination of the age of organic materials.
12. Advantages and Disadvantages of Radioactivity
As with any scientific marvel, the universe of radioactivity is a double-edged sword. On one hand, its applications in medicine are life-saving, providing valuable tools for diagnosis and treatment. Its ability to sterilize medical instruments and ensure the safety of consumable products is a boon to human health.
However, we must acknowledge the disadvantages and potential hazards associated with radioactivity. Prolonged exposure to ionizing radiation can have detrimental health effects, including radiation sickness and an increased risk of cancer. The disposal of radioactive waste poses environmental and safety challenges, necessitating robust protocols for containment and long-term management.
13. Nuclear Models and Decay Rates
In the quest to comprehend the intricate workings of radioactivity, physicists have devised nuclear models that provide insights into the stability and behavior of atomic nuclei. These models, such as the liquid drop model and the shell model, have proven instrumental in understanding the structure of atomic nuclei.
The concept of angular momentum conservation plays a pivotal role in elucidating radioactive transitions. Furthermore, the rate of radioactive decay exhibits variation based on the specific properties of isotopes, emphasizing the dynamic nature of nuclear physics.
14. Tropical Radioecology: Nature’s Radioactive Canvas
Overview of Natural Radioactivity – In exploring radioactivity, one cannot escape the pervasive influence of natural radioactivity. This phenomenon plays a profound role in geological processes, the evolution of life, and even the energy source that powers our planet.
Natural Radioactivity in Geological Processes – Radioactive elements, often called primordial or cosmogenic sources, significantly influence geological processes. From radiometric dating to the Earth’s internal heat, radioactivity is an intrinsic part of our planet’s story.
Connection Between Natural Radioactivity and Evolution of Life – The presence of radioactive isotopes in the environment has had a tangible impact on the evolution of life. By understanding these relationships, we unveil a deeper layer of the history of life on Earth.
Influence of Natural Nuclear Energy and the Sun– The Sun, our celestial energy source, relies on nuclear fusion for its existence. Understanding the processes that occur within the Sun’s core reveals the role of nuclear reactions in the celestial dance of stars.
15. Radioactivity in Coal and Coal-Related Compounds: Uncovering Hidden Transformations
Radioactivity in Coal and Pyrolysis Products – Coal and coal-related compounds are central to energy production and industrial processes. Yet, they harbor their own mysteries, including the distribution of 14C during pyrolysis.
Transformation of Naphthalene – Naphthalene, a common compound found in coal tar, undergoes a fascinating transformation process during pyrolysis. Unraveling this intricate process unveils the hidden world of radioactivity.
Mechanism of Pyrolysis and Formation of Coke – Pyrolysis, a fundamental process in the carbonization of coal, involves intricate chemical and radioactive transformations. Understanding these mechanisms is pivotal for energy and material production.
16. Progesterone Binding Proteins: Radioactivity at the Molecular Scale
Counting Procedures for Measuring Radioactivity – The investigation of radioactivity is not limited to macroscopic phenomena. At the molecular scale, **radioactive probe measurements** are employed to unveil the secrets of biological systems.
Identification of Radioactive Steroids – In the context of biological research, radioactive steroids are employed to uncover the interactions of hormones within living organisms, exemplifying the diverse applications of radioactivity.
Radioactive Probe Measurements and Analysis – Through precise measurements and sophisticated analyses, researchers shed light on the molecular-scale interactions that govern various biological systems, marking the union of radioactivity with biology.
17. Environmental Radioactivity: Navigating the Radiative Landscape
Introduction to Environmental Radioactivity – Our environment is permeated with radioactivity from various sources, both natural and artificial. Understanding environmental radioactivity is central to managing its effects and ensuring safety.
Ionizing Radiation Sources and Interactions – The universe is replete with ionizing radiation sources, from cosmic rays to terrestrial elements. Understanding their interactions with matter is essential for comprehending the impact of radiation on the environment and human health.
Role of International Organizations – Various international organizations, including the ICRP, UNSCEAR, IAEA, and NEA, play an essential role in regulating and guiding research on environmental radioactivity. These organizations ensure the responsible and safe use of nuclear materials.
18. Radioactivity and Nuclear Heating Measurements: Peering into Fusion Applications
Measurement of Induced Radioactivity – In the context of nuclear fusion, measuring induced radioactivity in various materials is paramount. This task is integral to ensuring the safety and feasibility of fusion as a clean energy source.
Discrepancies in Activation and Decay Data – Accurate measurement of activation and decay data is essential for safe nuclear fusion. However, discrepancies can arise due to complex nuclear reactions and the difficulty of precise measurement.
Nuclear Heat Deposition Rate Measurements – Understanding the nuclear heat deposition rate is essential for evaluating the performance and safety of fusion materials. This parameter dictates the feasibility of nuclear fusion as an energy source.
Discrepancies Between Measured and Computed Rates – The comparison between measured and computed rates reveals the complexities and challenges of nuclear fusion. This contrast highlights the intricacies of nuclear reactions and their implications for future energy solutions.
19. The Atomic Nucleus: Beta-Delayed Neutron Emission
Exploring Beta-Delayed Neutron Emission – The atomic nucleus is home to a diverse range of phenomena. Beta-delayed neutron emission is one such phenomenon that unveils a layer of complexity within the nuclear world.
Nuclides that Undergo Neutron Radioactivity – Certain nuclides have the remarkable capability to undergo **neutron radioactivity**, emitting neutrons as part of their decay process. Understanding these nuclides is pivotal for nuclear physics.
Position of Nuclides in the Chart of the Nuclides – The Chart of the Nuclides is a graphical representation of nuclear information, showcasing the unique features of each nuclide. Examining the location of nuclides that undergo neutron radioactivity provides insights into their behavior.
Measurement of Beta-Delayed Neutron Emission Probabilities – Accurate measurements of beta-delayed neutron emission probabilities are essential for nuclear reactors and the understanding of astrophysical phenomena. These measurements serve as cornerstones in nuclear physics research.
20. Bringing It All Together: The Complex Tapestry of Radioactivity
As we conclude our journey into the captivating world of radioactivity, we have unraveled the mysteries that span from the microscopic realm of atomic nuclei to the macroscopic landscapes of the environment. From the intrinsic nature of radioactivity to its vast implications, we’ve navigated a multidimensional universe that is both challenging and rewarding.
The allure of particle emissions, the enigma of decay modes, and the interplay of forces and nuclear reactions are threads that weave a complex tapestry of nuclear phenomena. We’ve ventured into the very essence of ionizing radiation, explored the fundamental processes of fission and fusion, and scrutinized the intricacies of induced and spontaneous radioactivity.
Our voyage into the depths of radioactivity has touched upon the cosmic origins of elements and the profound influence of radiation on life and geology. The atomic nucleus, with its unceasing disintegration and transformation, continues to be a source of unending fascination for scientists and enthusiasts alike.
Radioactivity, the product of the restless atomic nucleus, remains a captivating and integral aspect of the physical world. As we reflect on our journey, we can truly appreciate the depth and breadth of knowledge that radiates from this dynamic field. In both its fundamental principles and diverse applications, radioactivity is a testament to the power of human curiosity and the remarkable nature of the universe we inhabit.
You may also like to read:
The nuclear mass of 141ba is 140.883 amu. calculate the binding energy per nucleon for 141ba.