1. What is Gamma Decay?
Gamma decay is a type of radioactive decay in which an atomic nucleus emits a gamma ray, a high-energy photon with no mass or charge. Gamma decay is a result of an excited nucleus releasing energy in the form of a photon to return to a lower energy state. This process is often accompanied by other forms of radioactive decay, such as alpha or beta decay. The symbol for gamma is γ.
Gamma decay, a distinctive type of radioactive decay, sets itself apart by its emission of high-energy electromagnetic radiation – the elusive gamma ray photon. Unlike alpha or beta decay, no charged particles exit the nucleus during this process. Instead, the structure and composition of the atom remain unchanged, with only the energy of the atom undergoing modification. To comprehend gamma decay, envision protons and neutrons within the nucleus occupying discrete energy states. When a proton or neutron transitions from an excited state to a ground state, typically following an alpha or beta decay, it emits a gamma ray photon.
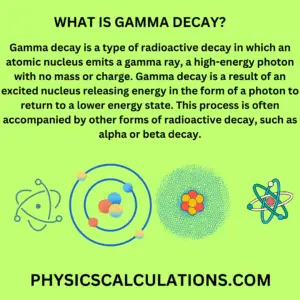
Explanation
Gamma decay is an essential process in nuclear physics that involves the release of γ-rays from an atomic nucleus. This type of decay is the most energetic form of radioactivity, as it involves the emission of photons with the highest energy level. Additionally, γ-decay plays a vital role in many areas, from medical imaging to nuclear power generation. In this article, we’ll take a closer look at γ-decay, including what it is, how it works, and its applications.
2. Types of Radioactive Decay
Before diving further into γ-decay, it’s important to understand the three main types of radioactive decay:
a. Alpha Decay
Alpha decay occurs when a radioactive nucleus emits an alpha particle, which consists of two protons and two neutrons, and has a charge of +2. We notice alpha decay in heavy elements, such as uranium and plutonium.
b. Beta Decay
Beta decay occurs when a radioactive nucleus emits a beta particle, which can either be an electron (β-) or a positron (β+). Hence, Beta decay happens when there are too many neutrons or protons in a nucleus, and the nucleus tries to achieve stability by converting one type of particle into another.
c. Gamma Decay
Gamma decay is a high-energy form of radioactivity that occurs when an excited nucleus releases energy in the form of a γ-ray. It often accompanies other forms of radioactive decay. Thus, it is the only type of decay that doesn’t involve the emission of particles.
3. The Nature of Gamma Radiation
Gamma radiation is a form of electromagnetic radiation, just like visible light and X-rays. However, gamma rays have a much higher energy level than other forms of radiation. This makes them extremely dangerous to living organisms. Additionally, the rays can penetrate most materials. These materials include concrete and steel. Therefore, they can cause the ionization of atoms and molecules, leading to cellular damage and radiation sickness.
4. Gamma Decay Process
Gamma decay occurs when an excited nucleus releases energy in the form of a γ-ray. Excited nuclei are created through various processes, such as nuclear reactions or other forms of radioactive decay. When an excited nucleus releases a gamma ray, the nucleus returns to a lower energy state, becoming more stable in the process.
5. Gamma Decay Equation
The gamma decay process can be represented by the following equation:
A* → A + γ
where A* is the excited nucleus, A is the daughter nucleus, and γ is the emitted gamma ray.
6. Half-Life of Gamma Decay
The half-life of a radioactive substance is the time it takes for half of its atoms to decay. Thus, γ-decay emits gamma radiation as a result of other forms of radioactive decay or nuclear reactions. They don’t have a specific half-life. However, scientists can measure the half-life of the excited nucleus before it undergoes γ-decay. Subsequently, they use it to calculate the probability of γ-decay occurrence.
7. Frequency Determination in Gamma Radiation
Understanding gamma radiation goes beyond acknowledging its occurrence; it extends to deciphering the frequency of the released gamma radiation. The equation Ei−Ef=hf unveils the intricacies of this frequency determination, where Ei represents the initial, higher energy state of the nucleon, Ef signifies the final, lower energy state, ℎ stands for Planck’s constant, and f denotes the frequency of the emitted radiation.
8. Applications of Gamma Decay
Despite their potentially harmful nature due to high energy, gamma rays emerge as invaluable tools in various applications. Their exceptional penetration capability makes them instrumental in medical procedures, particularly radiotherapy. Examples of significant gamma emitters include cobalt-60, cesium-137, and technetium-99m. These isotopes find applications in medical treatments, soil density measurement, oil well exploration, and irradiation of food for sterilization and preservation.
Gamma radiation also plays a pivotal role in enhancing the durability of materials. By toughening flooring in high-traffic areas, gamma rays contribute to the longevity of structures. Gamma decay has numerous applications in different fields, including:
a. Medical Imaging
Medical professionals use gamma rays for imaging techniques. These techniques include gamma cameras and PET (positron emission tomography) scans, to visualize the internal structures of the body. In gamma camera scans, the camera detects the gamma rays emitted by a radioactive substance introduced into the body to create images. Thus, to perform a PET scan, medical professionals inject a small amount of radioactive substance into the patient’s body. The substance emits positrons, which interact with electrons in the body, causing the release of gamma rays that the PET scanner detects
b. Industrial Radiography
In industrial radiography, professionals use gamma rays to examine materials for flaws or defects. They emit gamma rays onto the material using a gamma ray source and detect the gamma rays that pass through the material using a radiography film or detector. This process generates an image of the material’s internal structure.
9. Real-world Examples and Case Studies
Illustrating the practical applications of gamma decay, real-world examples and case studies offer insights into successful medical treatments, industrial breakthroughs, and the role of gamma decay in nuclear power plants. These instances serve as testaments to the versatility and significance of gamma radiation across diverse domains.
a. Nuclear Power Generation
Gamma rays are also a byproduct of nuclear power generation, where they are released during nuclear fission reactions. The gamma rays emitted during these reactions are used to heat water, which creates steam that drives turbines, generating electricity.
Gamma Decay in Nuclear Power Plants
In the field of nuclear power plants, uranium-238 and uranium-235 take center stage. These isotopes, integral to fueling nuclear reactors, undergo both alpha and gamma decays during the fission process. Immediate release of gamma rays post-fission raises radiation levels, necessitating stringent safety measures for workers’ protection.
10. Radiation Safety and Gamma Decay
Gamma radiation is extremely dangerous and can cause significant harm to living organisms. Therefore, it’s important to take necessary precautions when working with gamma radiation sources or in areas with potential gamma radiation exposure. These precautions include wearing protective gear, maintaining safe distances from radiation sources, and minimizing exposure time.
11. Future Developments in Gamma Decay Research
Research on gamma decay and its applications is ongoing, with scientists exploring new ways to harness the power of γ-radiation for medical, industrial, and energy applications. Advances in radiation detection and imaging technologies are also enabling more precise and efficient use of gamma radiation.
12. Conclusion
Gamma decay is a critical process in nuclear physics that involves the release of high-energy γ-rays from an atomic nucleus. This type of decay has numerous applications, including medical imaging, industrial radiography, and nuclear power generation. While gamma radiation is highly dangerous, proper safety measures can be taken to minimize the risks associated with working with gamma radiation sources.
You may also like to read: