1. What is Beta Decay?
Beta decay is a form of radioactive decay that occurs when a nucleus emits a beta (β) particle, which is either an electron or a positron. It occurs when there is an imbalance between the number of neutrons and protons in the nucleus of an atom. When this happens, the nucleus becomes unstable and emits a beta particle to regain stability. The symbol for beta is β.
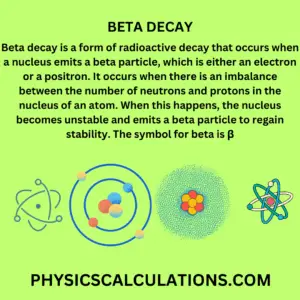
Understanding Beta Decay
Beta decay is a fundamental concept in nuclear physics and chemistry. In this process, a neutron or proton inside the nucleus of an atom transforms into an electron or a positron, respectively. Additionally, β-decay was first discovered in the late 19th century by French physicist Henri Becquerel.
Additionally, beta decay, a form of radioactive decay, involves the transformation of a proton into a neutron and vice versa within an atomic nucleus. This process is accompanied by the emission of beta particles, which can be either electrons or positrons. A crucial aspect of beta decay is its strict adherence to the law of conservation of charge, a fundamental principle governing the behaviour of particles in the subatomic realm. Furthermore, beta decay occurs through weak interaction, one of the fundamental forces that govern particle physics.
2. Types of Beta Decay
There are two types of beta decay: beta-minus and beta-plus. Beta-minus decay transforms a neutron in the nucleus into a proton and emits an electron and an antineutrino. While Beta-plus decay transforms a proton in the nucleus into a neutron and emits a positron and a neutrino.
To explain these two further, Beta decay manifests in two primary types: Beta-Minus and Beta-Plus. In Beta-Minus Decay, a neutron transforms into a proton, leading to an increase in the atomic number. This process produces an electron and an antineutrino, contributing to the delicate balance of charge within the nucleus. On the other hand, Beta-Plus Decay involves the transformation of a proton into a neutron, leading to a decrease in the atomic number. This process produces a positron and a neutrino, completing the beta decay repertoire.
3. Beta-Decaying Isotopes
We commonly observe β-decay in isotopes that have too many neutrons or too few protons to be stable. Some of the most common beta-decaying isotopes include carbon-14, tritium, and iodine-131.
4. Half-Life of Beta-Decaying Isotopes
The half-life of a β-decaying isotope is the time it takes for half of the radioactive material to decay into a more stable form. The half-life of beta-decaying isotopes can vary widely, from a few seconds to billions of years, depending on the specific isotope.
5. Beta Decay Equation
The β decay equation is a representation of the transformation that occurs during β decay. The general equation for beta-minus decay is:
n → p + e– + v
where n
represents a neutron, p
represents a proton, e-
represents an electron, and 𝜈
represents an antineutrino. The general equation for beta-plus decay is:
n → p + e+ + v
where e+
represents a positron and 𝜈
represents a neutrino.
6. Energy Release
Beta decay releases a significant amount of energy in the form of the kinetic energy of the β-particle and gamma radiation, which is a high-energy form of electromagnetic radiation.
7. Cracking the Code: Fermi’s Theory of Beta Decay
In 1933, Enrico Fermi introduced a groundbreaking theory to decipher the complexities of beta decay. According to Fermi, beta decay results from the direct interaction of four fermions: neutron, electron, neutrino (later identified as antineutrino), and proton. This elegant explanation became a cornerstone in understanding beta decay, providing a theoretical framework for the intricate dance of particles within the atomic nucleus.
8. Applications of Beta Decay
Beta decay has many applications in various fields, including medical imaging, radiocarbon dating, nuclear power, space exploration, agriculture, industry, and forensic science.
a. Medical Imaging
One of the most common applications of beta decay is in medical imaging. Positron emission tomography (PET) is a medical imaging technique that uses β-plus decay to produce images of the internal organs and tissues of the body. In PET, we inject a small amount of a beta-emitting radioisotope into the body, and as it decays, it emits positrons that combine with electrons in the body to produce gamma rays. The scanner detects these gamma rays and creates an image of the distribution of the radioisotope in the body.
b. Radiocarbon Dating
We also use β-decay in radiocarbon dating, which is a technique we use to determine the age of organic materials. Radiocarbon dating relies on the fact that carbon-14, an isotope of carbon, undergoes β-decay at a known rate. By measuring the amount of carbon-14 remaining in a sample, scientists can determine its age.
c. Nuclear Power
In nuclear reactors, beta-emitting isotopes produce energy by inducing a chain reaction of nuclear fission. Additionally, β-decay is also used to control the reaction rate by removing excess neutrons from the reactor.
d. Space Exploration
Additionally, β-decay is used in space exploration to power long-duration missions. Radioisotope thermoelectric generators (RTGs) use β-emitting isotopes to generate electricity by converting the heat generated by the decay of the isotope into electrical energy. RTGs, including the Voyager and Cassini spacecraft, have been used on several space missions.
e. Agriculture
Moreover, we also use β-decay in agriculture to measure the nutrient content of soils. In this application, we add β-emitting isotopes to soil samples and measure the rate of decay to determine the concentration of various nutrients, such as nitrogen and phosphorus.
f. Beta Decay In Industry
We apply β-decay in various industrial applications. These industries include thickness gauging and quality control. In thickness gauging, we use beta-emitting isotopes to measure the thickness of materials, such as metal or paper, by measuring the rate of β-particle transmission through the material. In quality control, we use β-decay to detect defects in materials, such as cracks or porosity.
g. Forensic Science
Moreover, β-decay is also used in forensic science to determine the age of samples, such as bones or teeth. Therefore, Like radiocarbon dating, this technique relies on the fact that some isotopes undergo β-decay at a known rate. By measuring the rate of decay of these isotopes in a sample, forensic scientists can estimate its age.
9. The Radioactive Ballet: What Happens in Beta Decay?
To comprehend beta decay, envision a subatomic ballet within the atomic nucleus. Here, a proton, positively charged, transforms into a neutron, neutral in charge, or vice versa. The nucleus releases high-speed beta particles, electrons, or positrons, creating a delicate equilibrium within the atomic dance. This equilibrium is maintained through the weak interaction, a force responsible for these transformations.
10. Example in Action: Beta Decay Case Study
Let’s examine beta decay in action, focusing on a common element: carbon. In this process, a carbon atom undergoes beta decay, resulting in the transformation of a neutron into a proton. The equation representing this decay provides a glimpse into the dynamic interplay of particles within the atomic nucleus, showcasing the real-world relevance of beta decay in isotopic transformations.
11. Beta Decay vs. Other Forms of Radioactive Decay
Beta decay is just one of the several forms of radioactive decay, which also include alpha and gamma decay. Alpha decay occurs when a nucleus emits an alpha particle, which consists of two protons and two neutrons. Gamma decay occurs when a nucleus emits a gamma ray, which is a high-energy form of electromagnetic radiation. Each form of radioactive decay has different characteristics and applications.
12. Comparisons and Context: Beta Decay in the Nuclear Landscape
Comparing beta decay with other forms of radioactivity, such as gamma and alpha decay, unveils distinctive characteristics. Unlike the relatively faster processes of gamma and alpha decay, beta decay occurs at a slower pace, with half-lives never shorter than a few milliseconds. This measured speed contributes to the uniqueness of beta decay in the intricate tapestry of nuclear reactions.
13. Trailblazers in Beta Decay Research
Acknowledging key figures in beta decay research is essential. Chien-Shiung Wu, Fermi, and Gamow-Teller have significantly contributed to unraveling the mysteries of beta decay. Their pioneering work paved the way for our current understanding, shaping the field of nuclear physics and influencing subsequent generations of physicists.
14. Conclusion
In conclusion, β-decay is a fundamental concept in nuclear physics and chemistry. It occurs when a nucleus emits a beta particle, either an electron or a positron, to regain stability. Hence, β-decay has many applications in various fields, including medical imaging, radiocarbon dating, nuclear power, space exploration, agriculture, industry, and forensic science. Understanding the basics of β-decay is crucial to understanding these applications and the role of radioactivity in our lives.
15. Frequently Asked Questions (FAQs)
- What is beta decay?
It is a form of radioactive decay that occurs when a nucleus emits a β-particle, which is either an electron or a positron, to regain stability.
- How does beta decay occur?
As we mentioned earlier, β-decay occurs when a nucleus has too many or too few neutrons in relation to its number of protons. During β-minus decay, a neutron converts into a proton and emits an electron and an antineutrino. Similarly, during β-plus decay, a proton converts into a neutron and emits a positron and a neutrino.
- What is the role of beta decay in medical imaging?
We can use β-decay in medical imaging techniques such as positron emission tomography (PET) to produce images of internal organs and tissues in the body.
- How is beta decay used in radiocarbon dating?
We also use β-decay in radiocarbon dating to determine the age of organic materials by measuring the amount of carbon-14 remaining in a sample.
- What is the difference between beta decay and alpha decay?
Alpha decay occurs when a nucleus emits an alpha particle, which consists of two protons and two neutrons. While β-decay occurs when a nucleus emits a β-particle, which is either an electron or a positron. Thus, both forms of decay involve the emission of particles from a nucleus to regain stability, but they differ in the nature and characteristics of the emitted particles.
You may also like to read: