1. What is Time?
We define time as the measure of the duration of events. We can equally define time as a continuous progression of events, marking the past, present, and future. It’s measured in units like seconds, defining the duration between occurrences. Time is a fundamental dimension that quantifies the progression of events, representing the intervals between occurrences and the duration of processes.
Additionally, it is a continuous and irreversible concept, providing a framework for measuring the sequence and duration of events in the past, present, and future. Time is often standardized using units such as seconds, minutes, and hours, facilitating consistent communication and coordination in various fields. The understanding of time is integral to physics, philosophy, and everyday life, influencing concepts of causality, change, and the organization of human activities. Time is perceived subjectively and experienced universally, serving as a crucial parameter in describing the temporal aspects of the universe.
2. Understanding Time in Physics
In physics, time is defined as a measurement of the duration of events. It is a way to describe how long something takes to happen. When we play soccer, we use time to measure how long the event needs to take place. Therefore, for us to measure time, we often use seconds, minutes, hours, and so on. Additionally, time is more than just a way to measure the duration of events. It is a fundamental concept that helps us to describe the behavior of the universe itself.
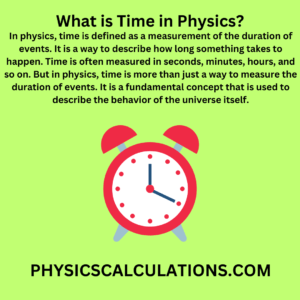
Have you ever stopped to think about what time really is? It’s a concept that we take for granted in our daily lives. However, in the world of physics, time is a fundamental concept that plays a crucial role in our understanding of the universe.
Time is a concept that has puzzled scientists and philosophers for centuries. We describe time as the fourth dimension, along with space. It is a fundamental concept that we use to describe the behaviour of matter and energy in the universe. But what is time, exactly? of course, the answer is the first paragraph of this article.
In this article, we’ll explore the concept of time in physics and try to answer some of the most frequently asked questions about it.
3. The Nature of Time in Physics
In physics, time is a scalar quantity. Hence, it can only have magnitude but no direction. It is also a continuous quantity. This tells us that it can be subdivided into smaller and smaller intervals without limit. However, this is in contrast to space, which is a vector quantity, with both magnitude and direction.
According to the theory of relativity, time is not absolute, but is relative to the observer. Thus, time can appear to pass differently depending on your frame of reference. For example, time can appear to pass more slowly for an object that is moving at a high-speed relative to an observer who is at rest. This is what we call time dilation.
4. History of time
The history of time is a multifaceted journey encompassing both cultural perceptions and scientific advancements. Early human societies observed time through natural phenomena, like the cycles of the sun and the moon, creating rudimentary calendars to track seasons and agricultural activities. Ancient civilizations, such as the Egyptians and the Babylonians, developed more sophisticated calendars based on celestial events.
In ancient Greece, philosophers like Parmenides and Heraclitus contemplated the nature of time, contributing philosophical perspectives that influenced Western thought. Aristotle introduced the concept of time as the measurement of motion, laying a foundation for future scientific understanding.
The medieval period saw advancements in mechanical timekeeping devices, including water clocks and mechanical clocks in towers. The pendulum clock, invented by Christiaan Huygens in 1656, marked a significant leap in accuracy. Concurrently, astronomical observations led to more precise calendars, addressing the inaccuracies of earlier systems.
The 18th and 19th centuries witnessed the standardization of timekeeping with the advent of chronometers, critical for navigation. The establishment of time zones in the 19th century, particularly at the International Meridian Conference in 1884, aimed to synchronize global timekeeping.
Einstein’s theory of relativity in the early 20th century fundamentally altered the understanding of time, introducing the concept of time dilation. The development of atomic clocks and the definition of the second based on atomic vibrations further refined precision.
Today, atomic time standards, including Coordinated Universal Time (UTC), govern global timekeeping. The quest for ever more accurate measurements continues, with developments in quantum timekeeping and the exploration of time’s role in cosmology and fundamental physics. The history of time reflects humanity’s evolving comprehension, blending cultural insights with scientific achievements.
5. The Units of Time
We measure time in seconds. However, there are other units of time that we use in certain contexts. For example, in astronomy, the unit of time used is often the year. We can explain this by saying that “the time it takes for the Earth to orbit around the Sun once”.
6. Time Standards in Relation to Physics
We will now clarify the terms and differences between UTC (Coordinated Universal Time), GMT (Greenwich Mean Time), and a few others:
Time Standard | Definition | Location | Difference from UTC |
---|---|---|---|
UTC (Coordinated Universal Time) | The primary time standard by which the world regulates clocks and time. | Worldwide | UTC is the reference point, and there is no difference. |
GMT (Greenwich Mean Time) | Originally referring to mean solar time at the Prime Meridian (0° longitude). | Primarily used in the UK, and as a historical reference. | Historically, GMT was used as the reference, but it’s now largely replaced by UTC. |
BST (British Summer Time) | The daylight saving time observed in the United Kingdom during the summer months. | UK | UTC+1 during BST. |
CET (Central European Time) | Standard time in the time zone that is one hour ahead of UTC (UTC+1). | Central European countries like France, Germany, Spain, etc. | UTC+1 |
CEST (Central European Summer Time) | The daylight saving time observed in Central European Time during the summer months. | Central European countries during summer. | UTC+2 during CEST. |
EST (Eastern Standard Time) | Standard time in the time zone that is five hours behind UTC (UTC-5). | Eastern United States during standard time. | UTC-5 |
EDT (Eastern Daylight Time) | The daylight saving time observed in Eastern Time during the summer months. | Eastern United States during daylight saving time. | UTC-4 during EDT. |
IST (Indian Standard Time) | The time observed throughout India and Sri Lanka, which is 5 hours and 30 minutes ahead of UTC. | India, Sri Lanka | UTC+5:30 |
JST (Japan Standard Time) | The time standard used in Japan, which is 9 hours ahead of UTC. | Japan | UTC+9 |
AEST (Australian Eastern Standard Time) | The time standard used in eastern parts of Australia, which is 10 hours ahead of UTC. | Eastern Australia | UTC+10 |
Please note that daylight saving time is not observed uniformly across the world, and some regions may not use it at all.
7. Definition of Second
The second is the base unit of time in the International System of Units (SI). It is defined as the duration of 9,192,631,770 periods of radiation corresponding to the transition between two hyperfine levels of the ground state of the cesium-133 atom. This definition, adopted in 1967, replaced an earlier definition based on Earth’s rotation.
The choice of the cesium-133 atom was driven by its remarkable stability, providing a highly accurate and reproducible time reference. The transition between hyperfine levels involves the emission or absorption of microwave radiation at a frequency of exactly 9,192,631,770 hertz.
The precision of atomic clocks, based on this definition, has revolutionized timekeeping. Atomic clocks are now the standard for measuring time, and they form the basis for Coordinated Universal Time (UTC), ensuring global synchronization.
The second is subdivided into milliseconds (1/1000th of a second), microseconds (1/1,000,000th of a second), nanoseconds (1/1,000,000,000th of a second), and further into smaller units for specific scientific or technological applications.
Advancements in atomic timekeeping, particularly with optical lattice clocks and other quantum technologies, continue to push the boundaries of precision. The understanding and measurement of the second are crucial in fields such as telecommunications, global navigation systems, scientific research, and numerous technological applications where precise and synchronized timekeeping is essential.
8. What is a Minute?
A minute is a unit of time equal to 60 seconds. It’s commonly used for precise measurement, scheduling, and expressing short durations. There are 60 minutes in an hour. The concept of a minute is fundamental in daily life, scientific measurements, and various timekeeping systems.
9. Hour, Day, Week, Month, and Year
Hour:
An hour is a unit of time equal to 60 minutes or 3,600 seconds. It divides a day into 24 segments. Hours are commonly used for daily scheduling and measuring short durations.
Day:
A day is the time it takes for Earth to complete one full rotation on its axis. It consists of 24 hours. Days are fundamental for organizing daily routines, astronomical observations, and various timekeeping systems.
Week:
A week is a unit of time consisting of seven days. It provides a practical way to organize time for work, religious practices, and social activities. The seven-day week has cultural and historical significance, and it forms the basis of many calendars.
Month:
A month is approximately the time it takes for the Moon to complete one orbit around Earth. Different calendars use varying definitions for months, but the average is close to 30 days. Months are fundamental in organizing longer durations, particularly in financial and calendar systems.
Year:
A year represents the time it takes for Earth to complete one orbit around the Sun. The most common calendar year is the Gregorian year, consisting of 365 days, with an extra day added every four years as a leap year. Years are crucial for agricultural planning, seasons, and long-term planning.
Conversion:
- 1 hour = 60 minutes = 3,600 seconds
- 1 day = 24 hours = 1,440 minutes = 86,400 seconds
- 1 week = 7 days
- 1 month ≈ 30 days (on average)
- 1 year = 365 days (common year), 366 days (leap year)
Conversion between these units involves simple arithmetic. For example, to convert days to hours, multiply the number of days by 24 (hours per day). Understanding these conversions is essential for coordinating activities, scheduling, and navigating various calendar systems. It also provides a framework for comprehending the different scales at which we measure and organize time in our daily lives and the broader context of Earth’s celestial motions.
10. What is a Decade?
A decade is a unit of time representing a period of ten years. It is derived from the Greek word “dekas,” meaning ten. This concept is widely used to analyze and describe historical, cultural, and societal trends over a specific span. The term can refer to any ten-year interval and we often use it in contexts such as decades of the 20th century or a decade in a person’s life.
a. Conversion of Decade to Smaller Units
To convert decades into smaller units:
- Years: One decade equals ten years. This conversion is straightforward, as a decade is precisely ten annual cycles.
- Months: A decade comprises 120 months. This conversion is based on the average of 12 months per year.
- Weeks: There are approximately 521.428 weeks in a decade, considering an average of 365.25 days per year.
- Days: A decade consists of roughly 3,652.5 days, accounting for leap years.
- Hours: There are around 87,660 hours in a decade, calculated by multiplying the number of days by 24 hours.
- Minutes: With 1,051,200 minutes in a decade, this conversion involves multiplying the number of hours by 60.
- Seconds: There are approximately 63,072,000 seconds in a decade, obtained by multiplying the number of minutes by 60.
The knowledge of the concept of a decade and its conversions is valuable for historical analysis, long-term planning, and discussions about societal and cultural shifts. It provides a framework for organizing and contextualizing events and trends over extended periods, offering insights into the evolution of various aspects of human life and civilization.
11. What is a Century?
A century is a unit of time representing a period of 100 years. The term is derived from the Latin word “centum,” meaning one hundred. Centuries are commonly used to frame historical eras, analyze long-term trends, and discuss the evolution of civilizations. The concept is crucial in various fields, including history, astronomy, and demography.
a. Conversion of Century to Smaller Units
To convert a century into smaller units:
- Years: One century is equivalent to 100 years, marking the completion of a centennial cycle.
- Decades: A century comprises ten decades, each spanning ten years.
- Months: There are approximately 1,200 months in a century, considering an average of 12 months per year.
- Weeks: There are about 5,243 weeks in a century, accounting for leap years.
- Days: A century consists of approximately 36,525 days, including leap years.
- Hours: There are around 876,600 hours in a century, calculated by multiplying the number of days by 24 hours.
- Minutes: With 52,596,000 minutes in a century, this conversion involves multiplying the number of hours by 60.
- Seconds: There are approximately 3,155,760,000 seconds in a century, obtained by multiplying the number of minutes by 60.
Understanding the concept of a century and its conversions is essential for historical analysis, demographic studies, and discussions about the long-term impacts of social, technological, and cultural changes on human civilization.
12. Time Standards Based on Earth Rotation
Time standards based on Earth’s rotation have a historical foundation and were essential for early timekeeping. The solar day, defined by one complete rotation of Earth on its axis relative to the Sun, initially served as the basis for time measurement. However, due to the irregularities in Earth’s rotation caused by factors like tidal forces, earthquakes, and atmospheric conditions, this method faced challenges in achieving precise and uniform timekeeping.
The Greenwich Mean Time (GMT), established in the 19th century, used the mean solar time at the Prime Meridian (located in Greenwich, London) as a reference. Despite its historical significance, GMT faced accuracy issues due to Earth’s variable rotation speed.
The Coordinated Universal Time (UTC) emerged as a compromise between atomic timekeeping and Earth’s rotation-based time. UTC is based on International Atomic Time (TAI), which uses a network of highly accurate atomic clocks. To account for irregularities in Earth’s rotation, leap seconds are periodically added to UTC, aligning it with the astronomical time based on Earth’s rotation.
While atomic time standards now dominate, Earth rotation-based time remains relevant in civil timekeeping. However, the increasing precision required for modern technologies has shifted the primary focus to atomic time standards. The development of International Celestial Reference Frame (ICRF) and International Terrestrial Reference Frame (ITRF) further refines the relationship between timekeeping and Earth’s rotational parameters. Despite the challenges posed by Earth’s rotation irregularities, these time standards remain crucial for astronomical observations, celestial navigation, and certain scientific applications.
13. Time Standards for Planetary Motion Calculations
Time standards for planetary motion calculations play a vital role in predicting celestial events and understanding the dynamics of our solar system. Unlike Earth-based timekeeping, these standards are tailored to the specific orbital characteristics of planets.
The concept of the sidereal day serves as a fundamental unit in planetary motion calculations. It is defined as the time it takes for a planet to complete one full rotation on its axis relative to a distant star. This definition eliminates the complications introduced by Earth’s own orbit around the Sun, providing a more stable reference for planetary observations.
In astronomical calculations, the Julian day is frequently employed. It is a continuous count of days since an initial epoch, enabling astronomers to express time consistently across different planetary positions and observations. The Julian day is particularly useful in predicting and analyzing events such as planetary conjunctions, oppositions, and transits.
For more accurate predictions, Ephemeris Time (ET) was introduced as a time scale based on the orbital motion of the Earth around the Sun. However, due to the unpredictable variations in Earth’s rotation, Ephemeris Time was later replaced by Terrestrial Time (TT) and Barycentric Dynamical Time (TDB), both of which account for the effects of relativity and the motion of the solar system’s barycenter.
The development of these specialized time standards enables astronomers and astrophysicists to precisely model and calculate planetary positions and motions, enhancing our understanding of the dynamic interactions within the solar system. These time standards provide a consistent framework for coordinating observations, making predictions, and conducting accurate simulations of celestial events.
14. Clocks
- Atomic Clock:
An atomic clock is a highly accurate timekeeping device that uses the vibrations of atoms as a reference. The most common type uses cesium-133 atoms or, more recently, other elements like rubidium or strontium. These clocks are crucial for maintaining precise time standards globally. - Clock Synchronization:
Clock synchronization involves aligning the timekeeping of multiple clocks to ensure they display the same time. This is essential for coordinated activities, data communication, and scientific measurements, often achieved using network protocols or specialized technologies. - Clock Signal:
A clock signal is a regular, repeating electronic pulse used to synchronize various components in electronic systems. In computing and communication, clock signals ensure that operations occur at the correct time, facilitating coordination and efficiency. - Epoch (Astronomy):
In astronomy, an epoch is a specific moment in time used as a reference for celestial observations. It helps define positions of celestial objects and is vital for coordinating observations and data analysis. - Frequency Standard:
A frequency standard is a device that produces a stable and precise frequency, often achieved using oscillators. Atomic clocks serve as primary frequency standards, crucial for various applications, including communication and navigation systems. - Radio Clock:
A radio clock receives time signals from a radio transmitter, often synchronized to atomic clocks. These clocks automatically adjust for accuracy, providing precise timekeeping. - Time in Astronomy:
Time in astronomy is a critical parameter for coordinating observations, calculating orbital positions, and studying celestial events. It often involves specialized time scales to account for relativistic effects and Earth’s rotation irregularities. - Time Signal:
A time signal is a broadcast signal containing accurate time information. These signals, often transmitted by national standards laboratories, help synchronize clocks and maintain consistent time standards. - Time Metrology:
Time metrology involves the precise measurement and study of time. It includes the development and application of standards, techniques, and instruments for accurate timekeeping. - Time Transfer:
Time transfer refers to methods used to synchronize time between different locations. This is crucial for maintaining coordinated time standards globally, especially in telecommunications and navigation.
a. Additional Topics:
- Timekeeping on Mars:
Adapting timekeeping to Mars involves adjusting for the longer Martian day, known as a sol. Mission planners and scientists use specialized timekeeping systems to account for the unique rotational characteristics of the Red Planet. - Orbital Period as Unit of Time:
In astronomy, the orbital period of celestial bodies, such as planets or moons, serves as a natural unit of time. It represents the time taken for an object to complete one orbit around another, providing a contextually relevant measure for astronomical phenomena.
15. How to Find Time in Physics
In physics, finding time involves using equations that relate other variables like distance, velocity, and acceleration. The basic time formula is (time=distance/velocity). If acceleration is involved, more complex equations like the kinematic equations may be used, such as (time= {final velocity-initial velocity} / acceleration). The choice of equation depends on the given information and what is being solved for. These equations help physicists calculate the time taken for an event, motion, or process and are fundamental in understanding the temporal aspects of physical phenomena.
16. The Role of Time
Time plays a key role in our understanding of the universe. It describes the behavior of matter and energy. It is also a fundamental concept in the theories of relativity and quantum mechanics. Without time, we would have no way to describe the duration of events or the motion of objects in the universe.
17. Frequently Asked Questions
Here are some of the most frequently questions about time, along with their answers:
Q: What is the relationship between time and space in physics?
A: We see time and space as two aspects of the same thing: spacetime. We can explain this by saying that space and time are interwoven and cannot be considered separately.
Q: Is time travel possible according to the laws of physics?
A: According to our current understanding of physics, time travel is not possible. However, some theories, such as those involving wormholes and time dilation, suggest that it may be possible under certain circumstances.
Q: Can we reverse time in physics?
A: According to the laws of physics, time is a one-way street. It can only move forward and cannot reverse. This is known as the arrow of time.
Q: What is the difference between absolute time and relative time in physics?
A: Absolute time is a concept that suggests that time exists independently of any observer or measurement. Relative time, on the other hand, is a concept that suggests that time is dependent on the observer’s frame of reference and the measurement.
Q: How does time dilation work in physics?
A: Time dilation is the phenomenon where time appears to pass more slowly for an object that is moving at a high speed relative to an observer who is at rest. This effect is predicted by the theory of relativity and has been observed in experiments involving atomic clocks.
Q: What is the concept of “proper time” in physics?
A: Proper time is a concept in relativity that refers to the time interval between two events as measured by an observer who is at rest relative to both events. Proper time is an important concept in understanding time dilation and other relativistic effects.
17. Summary
Finally, time is a fundamental concept in physics that describes the behavior of matter and energy in the universe. It is a scalar quantity. We measure time in seconds and we see it as relative to the observer’s frame of reference. The concept of time is essential to our under standing of the universe and is a topic that continues to fascinate scientists and philosophers alike.
You may also like to read: