1. What is Line Spectra?
Line spectra, also known as atomic spectra, are unique patterns of light emitted or absorbed by atoms. Unlike the continuous spectrum of white light, where all wavelengths are present, line spectra are characterized by the emission or absorption of discrete, well-defined frequencies. The result is a series of sharp, parallel lines in the spectrum. These lines are akin to the distinct notes of a musical scale, each corresponding to a specific energy transition within the atom. Alternatively, line spectra consist of a number of well-defined lines each having a particular frequency or wavelength or color. It’s important to know that only certain wavelengths of light are emitted. These wavelengths are different for different elements and compounds.
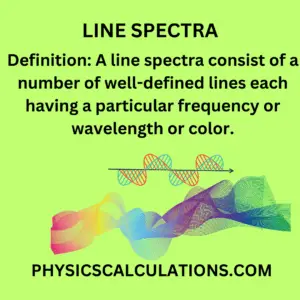
Additionally, the other names for Line spectra are atomic or discrete spectra. These are types of emission or absorption spectra that have only certain, discrete wavelengths. Their wavelengths correspond to the energy levels of the atoms or molecules that are emitting or absorbing the radiation. Each element or molecule has a unique line spectrum, which can be used to identify its composition and properties.
a. The Significance of Line Spectra in Spectroscopy
The study of line spectra is indispensable in the field of spectroscopy, which is the science of analyzing light to gain insights into the composition, structure, and behaviour of matter. Line spectra serve as the fingerprints of elements, allowing scientists to identify and characterize substances with remarkable precision. Whether in a laboratory on Earth or in the far reaches of the cosmos, line spectra enable us to explore and understand the fundamental building blocks of the universe.
b. What is a Spectrum?
To understand line spectra, we first need to understand what a spectrum is. A spectrum is the distribution of electromagnetic radiation emitted or absorbed by a substance over a range of frequencies or wavelengths. When this radiation is split into its component colors, it forms a spectrum. Subsequently, each color represents a different wavelength or frequency. The most familiar example of this is the visible spectrum, which ranges from red to violet.
2. Historical Background
To appreciate the significance of line spectra fully, it’s essential to trace their historical development. These spectral lines have intrigued scientists for centuries and have been pivotal in the evolution of our understanding of atomic behavior.
a. Early Observations of Line Spectra
The earliest observations of line spectra date back to the 17th century when Sir Isaac Newton passed sunlight through a prism, creating a continuous spectrum of colors. The journey into the world of line spectra began with the work of Joseph von Fraunhofer, a German optician, and physicist, in the early 19th century. Fraunhofer observed hundreds of dark lines in the solar spectrum, now known as Fraunhofer lines, which revealed that sunlight passing through the Earth’s atmosphere was selectively absorbed by certain elements.
b. Challenges Faced by Classical Physics
The existence of line spectra posed a significant challenge to classical physics. Classical physics, which dominated scientific thought in the 19th century, could not explain the existence of these discrete spectral lines. Instead, it predicted a continuous spectrum for all elements, in stark contrast to the observations.
This challenge laid the foundation for groundbreaking work in the field of atomic physics, particularly the development of the quantum mechanical model of the atom.
3. The Bohr Model: Unraveling the Mysteries of Line Spectra
The breakthrough in understanding line spectra came with Niels Bohr’s revolutionary model of the hydrogen atom. Bohr, a Danish physicist, introduced a radical concept that would fundamentally change our perception of atomic structure.
a. Niels Bohr and the Hydrogen Atom
Niels Bohr’s model of the hydrogen atom, proposed in 1913, was a pivotal moment in the history of atomic physics. Bohr introduced the idea that electrons orbit the nucleus of the hydrogen atom in quantized energy levels, also known as electron shells. This concept was in stark contrast to the classical model of atomic structure, where electrons moved in continuous orbits, much like planets around the sun.
b. Quantized Energy Levels
The idea of quantized energy levels was a key component of Bohr’s model. Electrons could transition between these energy levels by either absorbing or emitting energy in the form of photons (particles of light). The energy of these photons was directly related to the difference in energy between the initial and final electron states. This concept laid the foundation for understanding why line spectra are composed of distinct, sharp lines.
c. Circular Orbits and Electron Behavior
Bohr’s model described electrons as moving in circular orbits around the nucleus, similar to the planets’ motion around the sun. However, these orbits were quantized, meaning that electrons could only occupy specific, discrete orbits. As electrons moved from one orbit to another, they would emit or absorb energy, resulting in the emission or absorption of specific wavelengths of light.
This breakthrough explained why atoms produced line spectra, where only certain wavelengths of light were observed. The idea of quantized energy levels within the atom resolved the longstanding puzzle of spectral lines.
4. Emission and Absorption Spectra: A Tale of Atomic Behavior
Now that we understand the basics of the Bohr model and quantized energy levels, we can explore the phenomena of emission and absorption spectra, shedding light on the intriguing behaviour of atoms.
a. Emission Spectra
Emission spectra occur when atoms release energy in the form of light. This happens when electrons transition from higher energy levels to lower ones. The energy lost by the electron is emitted as a photon of light with a specific wavelength. As a result, emission spectra are characterized by bright lines at precise wavelengths, each corresponding to a specific electron transition.
b. Absorption Spectra
Conversely, absorption spectra are produced when atoms absorb light, causing electrons to transition from lower to higher energy levels. In this case, atoms absorb photons with energies matching the energy difference between these levels, resulting in dark lines in the spectrum. These lines are known as absorption lines.
Emission and absorption spectra are like two sides of the same coin, revealing different aspects of an atom’s behavior. While emission spectra display the wavelengths of light emitted by an excited atom, absorption spectra illustrate the wavelengths absorbed when an atom transitions to a higher energy state. Together, these spectra provide a comprehensive view of atomic behavior.
5. Line Spectra in Quantum Mechanics: The Quantum Leap
With a solid foundation in the Bohr model and the concept of emission and absorption spectra, it’s time to delve deeper into the realm of quantum mechanics, where line spectra find their true home.
a. The Quantum Mechanics Perspective
Quantum mechanics, a branch of physics developed in the early 20th century, provides a more comprehensive and accurate description of atomic behavior compared to classical physics. It is quantum mechanics that offers the most profound insights into line spectra and their origins.
b. Wavelengths and Energy Transitions
In the quantum mechanical view, line spectra are a result of quantized energy levels within the atom. As electrons transition between these energy levels, they emit or absorb photons of light. The energy of each photon is directly related to the energy difference between the initial and final electron states. This relationship explains why only specific wavelengths of light are emitted or absorbed, giving rise to the sharp lines in line spectra.
The understanding of line spectra in quantum mechanics represents a significant departure from classical physics, where electrons moved in continuous orbits, and a continuous spectrum was expected.
6. Beyond the Basics: Stark Effect and Quantum Insights
As we continue our exploration of line spectra and quantum mechanics, it’s essential to delve into more advanced concepts and phenomena that have expanded our understanding of atomic behavior.
a. The Stark Effect
The Stark effect is a phenomenon related to line spectra that was discovered by Johannes Stark in 1913. It involves the splitting of spectral lines in the presence of a strong electric field. This effect is the electric analogue of the Zeeman effect, which occurs in the presence of a magnetic field.
The Stark effect is a vivid demonstration of the profound connection between atomic behavior and external forces. In the presence of an electric field, the energy levels of the atom are shifted, leading to the splitting of spectral lines. This effect provides a powerful tool for understanding the interaction of atoms with electric fields and has profound implications for our comprehension of atomic structure and behavior.
b. Quantum Mechanics Advancements
The discovery of the Stark effect, along with other phenomena like the Zeeman effect and the Lamb shift, has opened new doors in the field of quantum mechanics. These phenomena demonstrate that the behavior of atoms cannot be fully explained by the classical models of physics. Instead, it requires the framework of quantum mechanics, with its probabilistic nature and quantized energy levels.
These advancements have not only deepened our understanding of atomic spectra but have also led to remarkable technological developments. Quantum mechanics, with its predictions and explanations for line spectra, has laid the foundation for numerous applications in science and technology, including the development of atomic clocks and precise measurement devices.
7. Practical Applications and Modern Significance
It’s essential to bridge the gap between theoretical concepts and real-world applications. The study of line spectra is not confined to the realm of abstract physics; it has practical implications that impact our daily lives.
a. Spectroscopy in the Real World
Spectroscopy, the science of analyzing light, has numerous practical applications. Whether it’s identifying the composition of distant stars, analyzing the elemental composition of a material in a laboratory, or determining the concentration of pollutants in the atmosphere, spectroscopy plays a crucial role.
By studying the line spectra emitted or absorbed by different substances, scientists and researchers can identify elements and compounds with exceptional accuracy. This capability has far-reaching implications in fields such as chemistry, astronomy, and environmental science.
b. Atomic Clocks and Beyond
One of the most remarkable applications of line spectra is in the development of atomic clocks. These clocks rely on the consistent and precise frequencies of atomic transitions to keep accurate time. The cesium atomic clock, for instance, uses the hyperfine transition of cesium atoms to define the international standard for the second.
Atomic clocks have revolutionized fields like global positioning systems (GPS), telecommunications, and space exploration. They provide the highly accurate timekeeping essential for synchronizing the operation of satellites, mobile networks, and scientific experiments. The importance of atomic clocks in our modern, interconnected world cannot be overstated.
c. Continued Exploration and Innovation
The study of line spectra continues to be a vibrant and dynamic field. Researchers are continually pushing the boundaries of our knowledge, aiming to overcome the limitations of existing models and develop new techniques for precise measurement. Whether it’s exploring the line spectra of complex molecules, studying the behavior of atoms under extreme conditions, or pushing the boundaries of our understanding of quantum mechanics, there are always new horizons to explore.
8. Types of Spectra
There are three types of spectra: continuous, emission, and absorption spectra.
- A continuous spectrum is a spectrum that has all wavelengths within a particular range. This spectrum is produced by a hot, glowing solid.
- An emission spectrum is a spectrum that has only certain wavelengths, or colors, present. For example, we have a spectrum of neon sign.
- An absorption spectrum is a spectrum that has certain wavelengths missing, or absorbed. Examples include the spectrum of sunlight that has certain colors missing due to absorption by the Earth’s atmosphere.
9. Limitations
While line spectra have numerous applications, they also have some limitations. One limitation is that they only provide information about the energy levels of the emitting or absorbing source. They don’t provide information for physical properties like temperature or pressure. Finally, the complex interactions between atoms and molecules make it difficult to interpret them.
10. Future
Despite its limitations, the line spectrum continues to be an important tool in our understanding of the universe. Advances in technology and theoretical models have allowed for even more precise measurements and interpretations of the line spectrum. In addition, the line spectrum has continued to be a source of inspiration for researchers. They gave birth to new discoveries and applications in fields ranging from materials science to quantum computing.
You may also like to read:
How to Calculate the Work Function of a Metal
Reference: